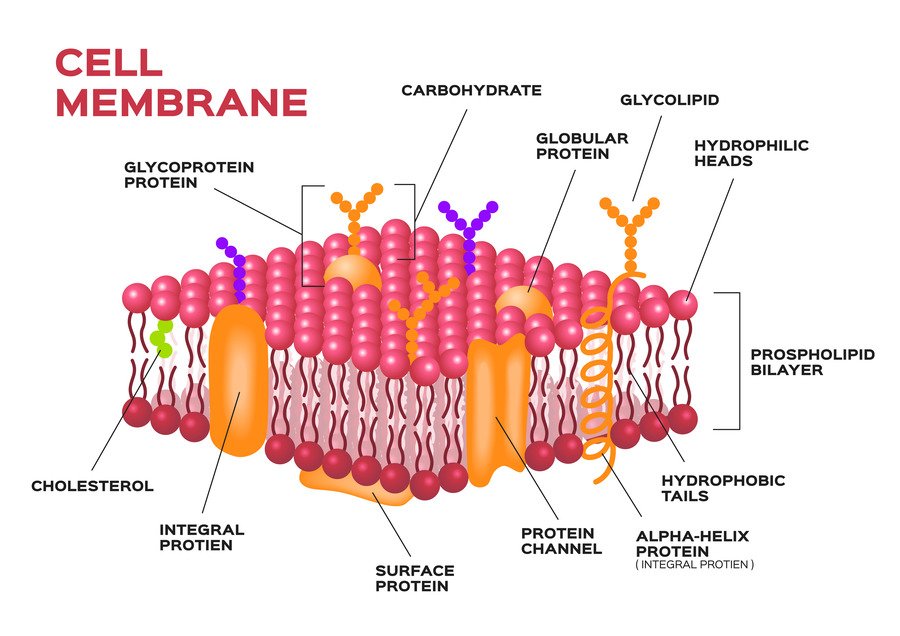
Cell surface glycosylation, the enzymatic process of attaching sugars to proteins and lipids, is fundamental to cell biology. Glycans, the carbohydrate moieties of glycoproteins and glycolipids, play critical roles in cell-cell communication, immune recognition, and pathogen interaction. Harnessing the specificity of glycosylation enzymes, chemoenzymatic cell surface labeling has emerged as a powerful tool for studying and manipulating cell surfaces. This blog post delves deeply into the world of glycosylation in chemoenzymatic cell surface labeling, exploring the enzymes involved, their mechanisms, and their diverse applications.
Understanding Glycosylation
Glycosylation involves the addition of oligosaccharides to proteins or lipids, resulting in the formation of glycoproteins or glycolipids. This process is catalyzed by a class of enzymes known as glycosyltransferases. Glycosylation can occur in various forms, including N-linked, O-linked, and C-linked glycosylation, each with specific structural and functional implications.
1. N-linked Glycosylation:
- Occurs in the endoplasmic reticulum and Golgi apparatus.
- Involves the attachment of oligosaccharides to the nitrogen atom of asparagine residues in proteins.
2. O-linked Glycosylation:
- Takes place in the Golgi apparatus.
- Involves the attachment of sugars to the oxygen atom of serine or threonine residues in proteins.
3. C-linked Glycosylation:
- Less common and involves the attachment of sugars directly to carbon atoms in amino acid side chains.
Glycosyltransferases in Chemoenzymatic Labeling
Glycosyltransferases are central to chemoenzymatic cell surface labeling. These enzymes exhibit remarkable specificity, recognizing distinct acceptor substrates and donor molecules, making them ideal for targeted modification of cell surfaces. Here, we discuss some key glycosyltransferases used in chemoenzymatic labeling:
1. Galactosyltransferases (GalTs):
- Function: Catalyze the transfer of galactose from a UDP-galactose donor to specific acceptor molecules.
- Example: GalT can be used to transfer galactose residues bearing bioorthogonal groups (e.g., azides or alkynes) to glycoproteins on the cell surface. These groups can then participate in click chemistry reactions for subsequent labeling.
2. Sialyltransferases (SiaTs):
- Function: Transfer sialic acid residues from CMP-sialic acid donors to terminal positions on glycan chains.
- Example: SiaTs can introduce sialic acids modified with bioorthogonal handles, enabling the selective labeling of sialylated glycoproteins.
3. Fucosyltransferases (FucTs):
- Function: Add fucose residues to specific acceptor sugars.
- Example: FucTs can attach fucose residues modified with chemical handles to glycans, facilitating their detection and analysis.
4. Glucosyltransferases (GlcTs):
- Function: Catalyze the transfer of glucose from UDP-glucose to acceptor molecules.
- Example: GlcTs can be used to introduce glucose moieties with reactive groups for downstream conjugation.
Mechanisms and Techniques of Glycosylation-Based Labeling
The process of chemoenzymatic cell surface labeling using glycosylation enzymes involves several key steps:
Substrate Recognition:
- Glycosyltransferases recognize specific glycan structures on the cell surface, ensuring targeted modification. This specificity is harnessed to introduce bioorthogonal groups only on desired glycoproteins or glycolipids.
Bioorthogonal Group Introduction:
- Modified sugar donors bearing bioorthogonal groups (such as azides or alkynes) are used. The glycosyltransferase transfers these modified sugars to the acceptor substrates on the cell surface.
Bioorthogonal Chemistry:
- The introduced bioorthogonal groups can participate in reactions such as azide-alkyne cycloaddition (click chemistry), Staudinger ligation, or tetrazine ligation. These reactions allow the attachment of various probes (e.g., fluorescent dyes) or functional groups (e.g., biotin) without interfering with native biochemical processes.
Optimization of Reaction Conditions:
- To achieve efficient labeling, reaction conditions such as pH, temperature, and enzyme concentration must be optimized. This ensures maximal activity and stability of the glycosyltransferase.
Applications of Glycosylation-Based Cell Surface Labeling
Glycosylation-based chemoenzymatic labeling has vast applications in biomedical research and therapeutic development. Here are some key areas where this technique is making significant impact:
Imaging and Visualization:
- Fluorescently labeled glycans enable high-resolution imaging of cell surface glycoproteins and glycolipids. This facilitates the study of cell surface dynamics, glycan distribution, and interactions in live cells.
Proteomics and Glycomics:
- Chemoenzymatic labeling helps identify and characterize glycoproteins and glycolipids. This is crucial for understanding the roles of glycans in health and disease, and for discovering biomarkers.
Cellular Engineering:
- Engineered cells with specific glycan modifications can be created using glycosyltransferases. These cells are valuable for studying glycan functions and for developing cell-based therapies.
Targeted Drug Delivery:
- Glycosylation-based labeling can be used to attach therapeutic agents to cell surface glycoproteins, enabling targeted delivery of drugs to specific cell types or tissues.
Vaccine Development:
- Modified glycans on the surface of cells or viruses can be used to develop vaccines that elicit strong immune responses. Glycosyltransferases facilitate the precise modification of these glycans to enhance immunogenicity.
Future Directions and Challenges
While glycosylation-based chemoenzymatic labeling offers numerous advantages, there are challenges and areas for future development:
Enzyme Engineering:
- Developing glycosyltransferases with broader substrate specificity or enhanced catalytic efficiency could expand the range of applications. Engineered enzymes may also offer improved stability under various conditions.
Donor Substrate Availability:
- Synthesizing sugar donors with bioorthogonal groups can be complex and costly. Developing more efficient and cost-effective methods for producing these donors is essential.
Complexity of Glycan Structures:
- The diversity and complexity of glycan structures on cell surfaces pose challenges for selective labeling. Advances in glycan engineering and better understanding of glycosyltransferase specificities are needed.
In Vivo Applications:
- Extending glycosylation-based labeling techniques to in vivo systems requires addressing issues such as enzyme delivery, substrate availability, and potential immunogenicity.
Conclusion
Glycosylation-based chemoenzymatic cell surface labeling is a powerful and versatile tool that leverages the specificity of glycosyltransferases to introduce bioorthogonal groups onto cell surface glycans. This technique has profound implications for imaging, proteomics, cellular engineering, and targeted therapies. As research advances, overcoming current challenges and refining these methods will unlock even greater potential for glycosylation-based technologies, driving new discoveries and innovations in cell biology and medicine. Embracing the specificity and efficiency of glycosyltransferases in chemoenzymatic labeling will continue to transform our understanding and manipulation of the cellular interface.